The 2023 Nobel Prize in Chemistry was awarded for the discovery and synthesis of colloidal quantum dots. Three scientists were awarded the prize: Aleksey Yekimov, Louis Brus and Moungi Bawendi.
What is so special about quantum dots?
These are nanoparticles (particles of the order of 10-9 metres in size, or one millionth of a millimetre) of semiconductor material. What makes them special? Their properties are determined by their size. This is highly unusual: the properties of materials are typically independent of their size. But it turns out that when the size is reduced to the nanometre scale, it is possible to obtain significant variations in electronic properties. This is called the phenomenon of quantum confinement. It’s important to understand that this property is truly incredible. Before their discovery, the only way to vary the properties of a material was to change its composition.
How is this property exploited today?
Essentially, they are used as light sources. Quantum dots are excited when exposed to light. They then return to their fundamental state by emitting a photon, an elementary particle of light. The colour of this photon depends very much on the size of the quantum dot. A process developed by Philippe Guyot-Sionnest, a former polytechnician, makes this photoluminescence process extremely efficient, with a yield close to 100%.
Are there any practical applications?
Yes, they can be found in QLED televisions. Blue diodes excite the screen’s quantum dots to generate the TV’s display. Compared with conventional technologies, colour purity is significantly improved. This is the main industrial application for quantum dots.
Other projects involve anti-counterfeiting devices. By integrating a mark using a quantum dot on the object to be certified, it is then possible to easily verify its presence using a light source. The advantage: this device is difficult to manufacture and easy to handle. Finally, new applications are emerging in the field of infrared detection. By depositing quantum dots (which absorb infrared light) on a conventional camera reading circuit, we can create an infrared camera. This technology considerably increases sensitivity. This application is still at the research stage, and several manufacturers, including the French company ST Microelectronics, are developing this type of camera.
Have scientists also adopted this technology?
Biologists were quick to embrace the subject. Quantum dots are used to study biological phenomena. How do they work? A biological species (such as a toxin) is attached to a quantum dot. The quantum dot is then placed in a growth medium containing cells. By illuminating the sample, it is possible to follow the trajectory of the toxin thanks to the luminescence of the quantum dot. The observation can be carried out over a long period, unlike the observation systems previously used. Maxime Dahan, a French biophysicist, has thus observed in vitro the phenomenon of information transmission at the synaptic level.
What are the benefits that are encouraging both industry and scientists to turn to these materials?
Quantum dots stand out from other materials in two respects. Firstly, it is possible to modulate their absorption and emission properties very precisely by modifying their size and chemical composition. This is a very interesting property for luminescence applications such as televisions: all you have to do is change the size of the quantum dots to control their emission colour. They can cover a very wide range of wavelengths, from 400 nanometres to a few microns (visible and infrared light).
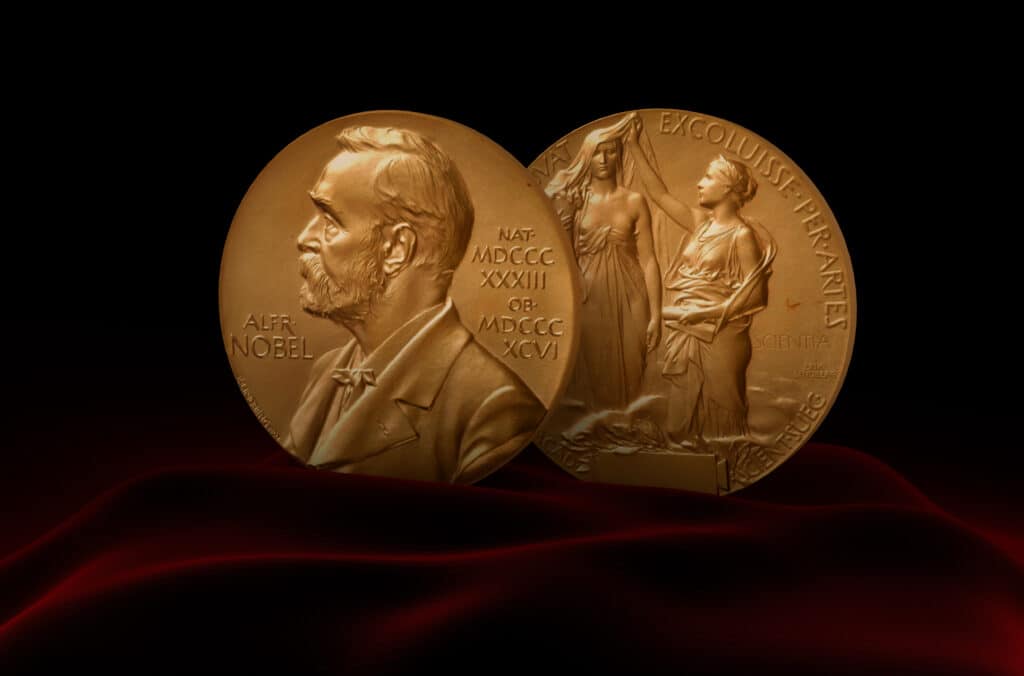
In addition, these are inorganic materials, which gives the signal stability. The only shortcoming is the blinking effect of the quantum dots. But it is now possible to overcome this by using more complex synthesis techniques.
How easy is it to manufacture quantum dots?
The launch of QLED TV sets is proof that it is possible to manufacture them on an industrial scale. Their synthesis is not a simple matter. The difficulty lies in controlling the size of the particles. Their size – on a nanometric scale – is mainly controlled by the temperature at which they are formed. On an industrial scale, it is therefore necessary to maintain a perfectly homogenous temperature in large-scale reactors.
Thanks to the work of Aleksey Yekimov and Moungi Bawendi, two of the three Nobel Prize winners, we are now at the stage of industrial production. The method they developed revolutionised the chemistry of nanocrystals and is now used to synthesise many other materials such as iron oxide, tungsten and titanium.
Can you retrace the history of this Nobel Prize-winning discovery?
This research began in the early 1980s with the first experimental observations. Aleksey Yekimov observed the variation in the spectrometric properties of coloured glass as a function of the heat treatment of the material. He was the first to make the connection between the size of the small semiconductor precipitates he observed in glass and its properties. This is a marked phenomenon in glass, as it is visible to the naked eye: when heat annealed at between 250°C and 400°C, a colour gradient is observed, from yellow (small semiconductor crystals in the glass matrix) to red (large semiconductor crystals). Louis Brus was the first to explain the physics behind the observed phenomenon known as quantum confinement, by Aleksey Yekimov.
Moungi Bawendi, a student of Louis Brus, developed an advanced synthesis method. It was proving difficult to precisely control the size distribution of glass particles, and therefore the properties of the material. Moungi Bawendi came up with the idea of manufacturing crystals in colloidal suspension, i.e. in a solvent. He mixes precursors (cadmium and selenium) in a solvent, leading to the formation of cadmium selenide crystals. By carrying out this synthesis at high temperature (250–300°C), the nucleation and growth of the crystals are very well controlled. This is the key to controlling the size and distribution of particles, and therefore their properties. His work has revolutionised the field of crystal production using colloid chemistry.
Could there be other areas of application in the future?
This remains a particularly active field. Chemists are continuing to improve materials and to propose new strategies for the emergence of interesting properties: applications in catalysis, in photocatalysis for artificial photosynthesis, the assembly of nanocrystals to form supracrystals with new collective properties, etc. Research teams are also working on the shape of quantum dots, making rods rather than spheres. This could pave the way for new applications in biology to better characterise the flow of fluids such as blood. Physicists, for their part, are adopting them because of their ultra-pure light-emitting properties: research is looking into the use of quantum dots in quantum computing and quantum cryptography. What’s more, thanks to their great flexibility and robustness, these quantum dots could become building blocks for nanotechnology.