The first weather forecasting models were developed in the early 20th century1 with the idea that if the initial state of the atmosphere – that is, the conditions of wind, humidity, temperature and pressure at a given time – are known precisely, then its future state can be predicted thanks to the equations of physics governing the temporal evolution of the atmospheric variables. For a weather forecast to be useful, it needs to be of a certain quality and provide predictions for the short term: it can therefore be thought of as a kind of race against time. Moreover, as the weather forecast at a given point depends on the weather conditions observed elsewhere, weather forecasters set up collaborations early on in the history of the field to exchange their observational data in real time and compare the performance of their models.
Ground and upper air observations
The first observational systems consisted of networks of instruments that measured atmospheric conditions in situ (at the exact location of the sensor) at ground level, but meteorologists soon realised that they also needed observations at altitude. They thus developed radiosondes – balloons carrying instruments to measure temperature, pressure, humidity, and wind, launched several times a day and reaching up to 20 km in altitude. Some balloons can also be launched from ships.
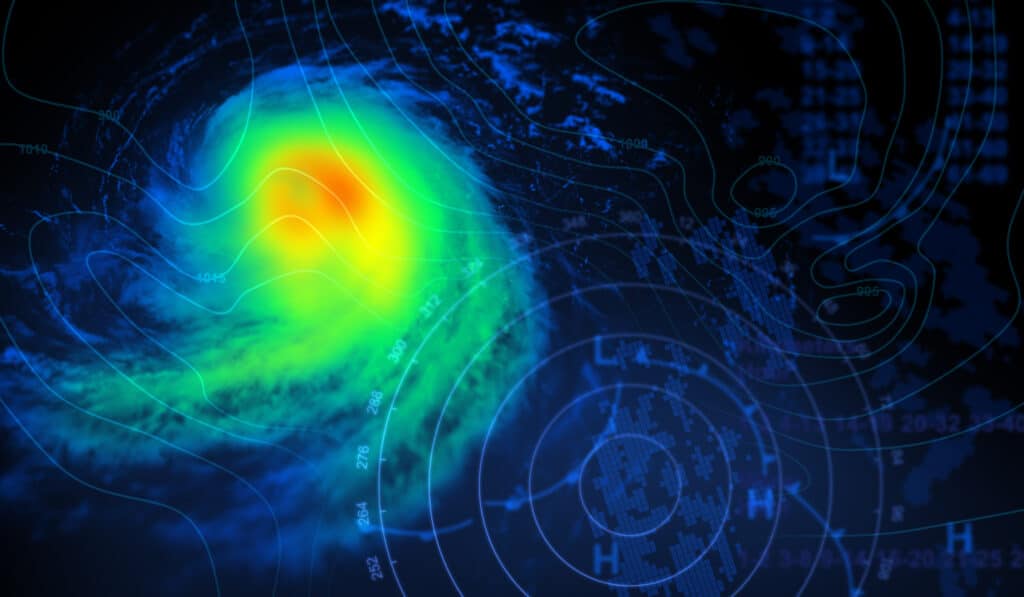
Observation technologies have evolved considerably and we are now witnessing the emergence of observation projects based on drones, which could be of operational interest for sampling the atmosphere at altitude, particularly over the sea (where deployment conditions are less constrained), following sampling strategies that are determined by meteorological conditions.
The advent of remote sensing
From the 1960–70s, remote sensing (the remote measurement of atmospheric parameters) began to be introduced into observation networks. Weather radar is an example of such remote sensing instruments. These devices emit electromagnetic waves that propagate and interact with rain, snow and hail, and can thus be used to map precipitation over areas of several hundred kilometres or more when the instruments are networked. Today, about 200 weather radars are in operation in Europe. The number is similar in the United States and Japan. For many years, weather radar data have been assimilated into numerical weather prediction models, significantly improving the quality of precipitation forecasts2.
Another example of a remote sensing instrument is lidar, which is similar to radar except that the waves emitted are light waves. These waves are sensitive to aerosols – small dust particles suspended in the atmosphere – or cloud droplets. Lidars can therefore measure the properties of these particles from a distance and are now part of the operational networks used by meteorological services3.
Satellite observations
Another major advance has of course been the emergence of satellite observations, which make it possible to observe large areas with the same instrument. Satellites can be divided into two main families. The first are the geostationary satellites, which remain permanently above a single point on the equator (at an altitude of about 36 000 km). As they can only observe half the globe, international collaboration is required to cover the whole planet.
The second type of satellite is the polar-orbiting satellite, which, as its name suggests, continually orbits the Earth (at an altitude of between 300 and 800 km), typically circling the globe in about 100 minutes. These satellites can provide observations at all latitudes, including the poles, with the same spatial resolution, unlike geostationary satellites. And because they are about ten times closer to the Earth, they offer better resolution of clouds and surfaces.
A wide variety of instruments operating in different wavelengths are carried on these satellites and allow the properties of the atmosphere to be measured: clouds, precipitation, aerosols, wind, temperature and humidity.
A new generation of European geostationary satellites has been launched.
The number of meteorological satellites has increased dramatically in recent years and ambitious programmes, particularly in Europe, have been launched. Last December, for example, a new generation of European geostationary satellites was launched4, as part of the European Agency’s programme for operational weather satellites.
Timely observations
Finally, there are the so-called timely observations – that is, those that come from an infrastructure that was not originally designed to carry out atmospheric measurements. An important example: mobile phone networks. These networks use antennas (there are several thousand of them in France) that communicate with each other at microwave wavelengths. Operators quickly realised that the level of reception between antennas was reduced when there were areas (or “cells”) of rainfall between them. This is because microwave wavelengths are affected by precipitation.
« Operators reacted to this problem by equipping the systems with a capacity to increase reception levels in the event of observed attenuation, but for meteorologists, the measurement of this observed attenuation was very interesting because it provided information on the intensity of precipitation occurring in a given area, » explains Pierre Tabary, Deputy Director of Operations at Météo France. This indirect information, properly processed, can help to improve precipitation maps5. « Nobody had imagined at the outset that mobile phone networks could be used in this way.
Positioning satellites – the GPS system in the US and Galileo in Europe – are another example of a timely-based measurement. « These dedicated positioning satellites are constantly emitting signals. So, the ingenious idea was to put other, much smaller satellites, that opportunistically pick up these signals, into orbit. The signals that are detected are slightly refracted on their way from the transmitting satellite to the receiving satellite as they pass through the atmosphere, so that they are somewhat ‘bent’. The level of this bending can be quantified by the receiving satellite and indirectly provides valuable information about thermodynamic conditions, such as humidity, in the stratosphere and upper troposphere.
Researchers have verified the relevance of this measurement principle (called radio occultation) and there are now several dozen reception satellites of this type in service, the data from which are analysed by operational weather forecasting models6. « Here again, we are able to carry out measurements at lower cost: we do not emit waves into the atmosphere ourselves, but we exploit the waves already emitted by others.”
Today, about 90% of the data input into global weather forecasting models comes from satellites and this trend will continue. « That said, ground-based measurements will always be important – especially for calibrating satellite data, » concludes Pierre Tabary.