As the regulation of genetic modification is changing in European Parliament1, the CRISPR-Cas9 gene-editing technology divides opinion. A source of concern for some, full of promise for others, let’s take a look at how it works, the possibilities it opens up, its limits and the questions it raises.
CRISPR-Cas9, the fruit of fundamental research
CRISPRs (Clustered regularly interspaced short palindromic repeats) are DNA sequences found in the genomes of many prokaryotes, both bacteria and archaea. First identified in Escherichia coli in 19872, they have a characteristic structure: short, conserved repeats are separated by other short, variable sequences known as spacers. It took a great deal of research over 20 years3 to understand that these are defence elements against viruses4.
Indeed, spacer sequences are portions of viral genomes, integrated by prokaryotes into their own genome. CRISPR DNA is transcribed into RNA, which in turn is cut to form small guide RNAs called crRNAs (CRISPR RNAs), derived from each of the spacers and therefore similar to viral sequences. When a virus corresponding to one of these spacers infects the cell, the associated crRNA binds to its genome. The structure thus formed is then targeted by an enzyme of the Cas family (for CRISPR-associated), capable of cutting the viral DNA, thus putting an end to the infection.
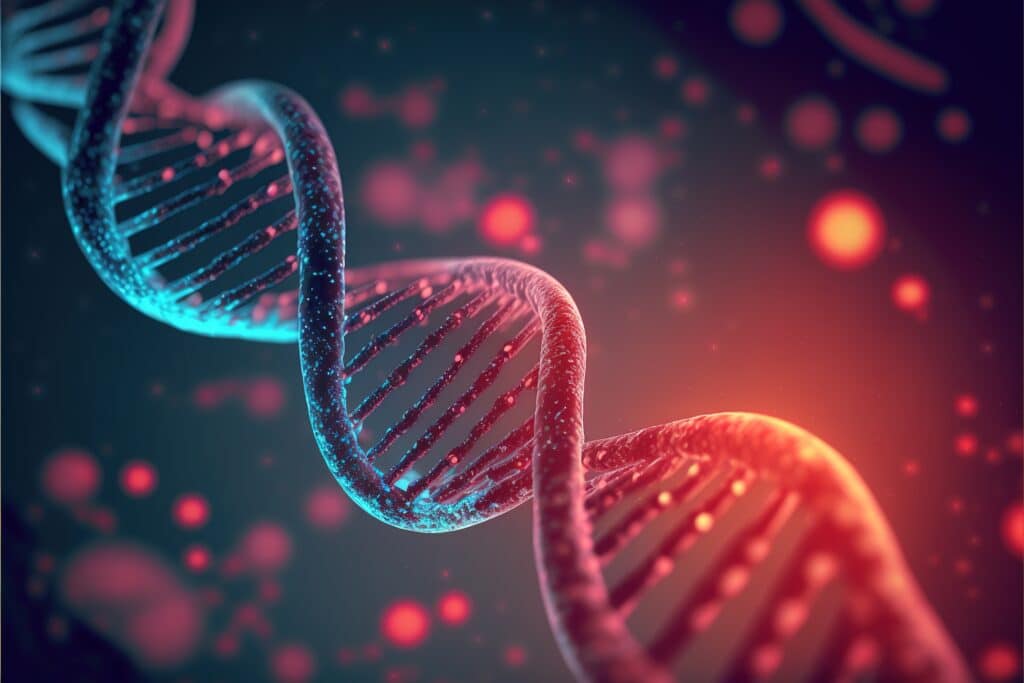
The CRISPR-Cas system therefore works in three stages: expression of crRNAs, recognition of the viral genome by a crRNA, and cutting by a Cas protein, guided to the right place by the crRNA. When a prokaryote encounters a new virus, it can recover a piece of its genome and add it to its CRISPR library: the CRISPR-Cas immune response is therefore specific to each virus encountered. What’s more, this memory is transmitted hereditarily, since it is stored directly in the genome. If this remarkable defence mechanism is now known well beyond the microbiology community, it’s because it has been adapted to design a particularly easy-to-use gene-editing tool.
Modifying genomes with CRISPR-Cas9
The general idea is to use the molecular scissors known as Cas enzymes to cut DNA where you want it, using a custom-designed crRNA to recognise a specific genetic sequence. Once the DNA has been cut, several things can happen: if the cell is left to repair the break, the sequence is altered in the process. But if it is provided with a DNA model, containing a relevant sequence (bordered by the same motifs as those found on either side of the break), this is used by the cell in a repair process called homologous recombination, a kind of molecular copy-and-paste. The DNA model sequence is integrated into the genome at the point where the cut was made.
By cutting where you want to insert any DNA sequence, the CRISPR-Cas9 technique makes it possible to modify genomes with extreme precision. This makes it possible to introduce small mutations into the DNA, add new genes or, if no repair model is provided, deactivate a gene. As it is now easy and inexpensive to order customised nucleic acids for use as crRNA, this method of gene editing is very accessible, as well as being highly effective.
By cutting where you want to insert any DNA sequence, the CRISPR-Cas9 technique makes it possible to modify genomes with extreme precision.
In prokaryotes, there are a wide variety of Cas proteins with different functions and characteristics5. The first gene-editing systems were developed with Cas9, which produces double-strand DNA cuts. However, several versions of this enzyme, isolated from different micro-organisms, recognise sequences of slightly different lengths with varying specificities. Other Cas enzymes are of particular interest, notably Cas12, which functions even more simply than Cas9. It shows promise for simultaneously modifying several sequences, for acting on the epigenome [editor’s note: all epigenetic modifications (modifying the expression of a gene) in a cell] and as a DNA detection system, particularly for diagnostic tests. Cas13 cuts RNA rather than DNA, making it an interesting tool for detecting RNA, including infectious RNA, and opening up the possibility of acting on the transcriptome [editor’s note: all the RNA resulting from the transcription of the genome present at a given moment], the result of the expression of a genome, without modifying the genome itself.
The CRISPR-Cas gene-editing field is currently booming, mobilising a large number of research teams. Pioneers Emmanuelle Charpentier and Jennifer Doudna were awarded the Nobel Prize in Chemistry in 2020 for their development of gene editing using CRISPR-Cas96, following a seminal paper they published in 20127. At the same time, other teams published the first results of eukaryotic cell genome modifications using CRISPR-Cas9 technology, including those led by Feng Zhang8 and his collaborator and former mentor, George Church9. These four scientists have since founded biotechnology companies focused on gene editing, and their supervisory institutions are waging a veritable patent war. Because the possibilities opened up by CRISPR-Cas technology have major financial implications.
The many applications of CRISPR-Cas
Developed just ten years ago, the CRISPR-Cas approach has already become a standard in fundamental biology research laboratories. Its speed and accessibility make it an ideal tool for switching genes off or modifying them, enabling a better understanding of their functions. It is now possible to carry out screens on an entire genome relatively easily. But the fields of application are much wider, and the boom is particularly marked in three areas: health, agronomy and bioproduction.
The ability to easily modify genes is particularly promising for treating monogenic diseases, i.e. those caused by the malfunctioning of a single gene. CRISPR-Cas9 makes it possible to envisage a new form of gene therapy, ensuring the lasting replacement of a mutated allele by a functional version. The first CRISPR-Cas therapy to be approved by the health authorities at the end of 2023 concerns genetic diseases. Casgevy, produced by Vertex and CRISPR Therapeutics and now approved in the United States and the United Kingdom, targets forms of chronic anaemia caused by a mutation in one of the haemoglobin genes. In France, this treatment has benefited from the early access authorisation exemption since 18th January 202410.
CRISPR-Cas could make it easier to treat diseases caused by the malfunctioning of several genes, but it cannot overcome all the difficulties posed by gene therapies. While certain organs such as the blood, eye and liver are easily accessible, delivering the treatment to all the cells that need it without causing side effects is still a challenge. However, the potential applications of CRISPR-Cas in human health are not limited to gene therapies and diagnostic tools. Trials are underway, for example, to try to eliminate HIV from the bodies of infected people11, to combat malaria12, to facilitate organ transplants13, to develop new approaches to diabetes14 and many others.
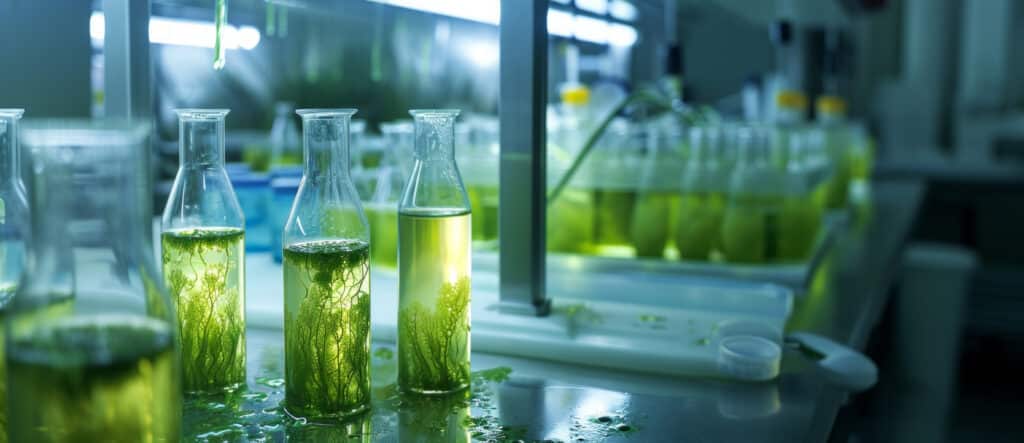
Since their invention, agriculture and livestock breeding have been based on the genetic modification of species in order to develop characteristics of interest to humans. This process, which involved centuries or even millennia of selection and cross-breeding, has been turned on its head by the development of molecular biology. Gaining a better understanding of the role of each gene and being able to modify them – or even insert new ones – in a precise and controlled way, instead of selecting random mutations a posteriori, represents a considerable gain in efficiency. What’s more, the potential applications are numerous. Adapting the varieties cultivated to heat, drought or other environmental factors, improving resistance to certain pests (to reduce the use of pesticides), increasing yields to reduce the need for fertilisers, extending the post-harvest shelf life of products to avoid wastage, improving nutritional qualities15… Once again, it is impossible to draw up an exhaustive list.
The targeted modifications made possible by CRISPR-Cas also make it possible to envisage new developments such as the production of naturally decaffeinated coffee16 or allergen-free foods. Attempts are underway, for example, to produce gluten-free wheat17. Conversely, CRISPR-Cas can be used to develop new bioproduction systems, by inserting the necessary genes into the genome of micro-organisms such as yeast, algae or bacteria. Biofuels, pharmaceutical compounds, cosmetics, food molecules such as vitamins: the possibilities are numerous and the ease of use of CRISPR-Cas is also revolutionising synthetic biology.
The limitations of CRISPR-Cas
CRISPR-Cas is sometimes presented as a kind of magic wand, but this gene-editing tool has its limits. It can be used to modify genomes precisely, but not to bring about just any change in the phenotype of an organism. Complex biological processes involving numerous genes cannot be easily reprogrammed, even with CRISPR-Cas. Genes, which are essential to the proper functioning of an organism, cannot be modified without harmful consequences. Even if a gene conferring a given property is known, it cannot be acquired by a new organism using CRISPR-Cas. And there is nothing obvious about transferring laboratory protocols (carried out under controlled conditions with often simplified systems) to real-life situations.
What’s more, while CRISPR-Cas editing technology is particularly precise, it is not 100% reliable. The appearance of so-called off-target modifications, other than at the level of the sequence initially targeted, is a problem for the therapeutic use of CRISPR-Cas. Other technical limitations remain to be overcome18. The accessibility of potential treatments based on CRISPR-Cas is also a point of concern. The price of Casgevy has been set at $2.2 million. Even if a single intervention were sufficient to cure patients, the use of such an expensive therapy is bound to remain restricted and uneven.
While some uses of CRISPR-Cas seem promising or anecdotal, others raise more difficult ethical questions.
This is just one of the many ethical issues raised by CRISPR-Cas technology. Like any tool, neither good nor bad in itself, it is its potential uses that need to be examined. Those that seem accessible in the near future, but also those that could become so in the longer term. Modifying the genome of sick people to cure them of an otherwise incurable disease seems promising, but touching the human genome is far from harmless. A Chinese scientist who genetically modified several embryos in an attempt to make them resistant to HIV was condemned by both his peers and the courts: he spent three years in prison before being released in 2022. The fact remains that he was able to produce several genetically modified babies as part of a project whose methodology was as flawed as its ethics19.
So while some uses of CRISPR-Cas seem promising or anecdotal, others raise more difficult questions. Is it acceptable to genetically modify plants to make them more resistant to drought? Is it acceptable to genetically modify cats to make them less allergenic20? Mosquitoes transmit diseases responsible for hundreds of thousands of deaths every year, but would it be acceptable to use a genetic forcing approach, based on CRISPR, to wipe out entire populations of mosquitoes21? If you don’t answer these three questions in the same way, what are the factors that sway your opinion?
These are complex questions, but given the speed at which technological tools are advancing, it is becoming imperative to answer them collectively. The G6 brings together six major European research organisations, including the CNRS. On the occasion of the recent debates in the European Parliament, it published a press release calling for risk assessment to be “based on the characteristics of genetically modified organisms, rather than on the techniques used to obtain them.”22 On this basis, what regulations might be laid down?