Quantum physics has now largely become part of our everyday lives. The “first quantum revolution” led to a host of devices and techniques that we use almost every day. Lasers, electronics, LED lighting, photovoltaic panels, nuclear medicine – none of these everyday technologies could be used without a detailed knowledge of the processes that take place on an atomic level, thanks to an understanding of the behaviour of elementary particles and the interactions between matter and light.
But quantum mechanics has not finished changing our world. The very latest discoveries from research laboratories foreshadow a second quantum revolution, in which mastery of the processes at work in the infinitesimal may once again profoundly change the way we live, communicate, and understand the world. Let’s take a look at the next applications of quantum physics in the industrial world.
Spintronics: the electronics of the future
The ever-finer control of electron flows in ever-smaller devices has enabled electronics to reach unprecedented levels of miniaturisation. In 2021, IBM announced the development of a chip made of 2 nanometre transistors, with a density of 333 million transistors per mm².
But in addition to its electrical charge, the electron has another property called “spin”. This quantum quantity has no classical equivalent but can be compared to a “magnetic moment”, as if the electron were a tiny magnet rotating on itself. The principle of spintronics is therefore to manipulate the spin of electrons rather than their electrical charge in order to create new applications, but also to dramatically reduce the power consumption of components.
Spintronics is already being used in a number of electronic components, including computer memory (for which its discoverers won the Nobel Prize in Physics in 2007) and certain magnetic sensors for cars and robotics.
Spintronics is already used in a number of electronic components, such as computer memories.
But, as mentioned above, the miniaturisation of electronic devices is so advanced that their basic elements will soon be the size of just a few atoms. A size that will make their behaviour almost exclusively quantum. To read and write on such small memories, a team from the Institut Rayonnement-Matière de Saclay (IRaMiS) has studied the behaviour of a molecule (called FeTTP) that normally plays a role in the transport of oxygen by haemoglobin1.
This molecule deposited on graphene (a layer of carbon atoms one atom thick) can change spin easily and at will. This constitutes a new read/write mechanism for a single molecular spin, which is even smaller and more energy-efficient than existing devices.
Meanwhile, the Nanosciences and Nanotechnologies Centre at the University of Paris-Saclay is trying to make artificial intelligence systems more flexible2. In conventional computer systems, the basic information is coded in the form of 0 or 1. New spintronic systems make it possible to introduce nuances into the binary code, such as 0+ or 1- states, and to integrate this ‘fuzzy’ logic into artificial neural networks, which would operate more like the organic biological neurons in our brains.
Quantum sensors: measuring the immeasurable
A large number of sensors are built around different quantum phenomena that give them the ability to measure minute signals with excellent resolution, opening up new fields of application.
In a microscope, the resolution limit is determined by the properties of the light used. Generally speaking, it is not possible to ‘see’ an object smaller than one wavelength of this light. In the visible range, this wavelength is around 500 nanometres.
In quantum physics, there is a principle called “wave-particle duality”, according to which quantum objects (particles, atoms, etc.) exhibit the behaviour of both particles and waves. We can therefore associate them with a wavelength, like light, and imagine a “matter wave microscope”. The advantage is that the wavelength associated with atoms is 1 million times shorter than that of light. We therefore have a measurement capability that is 1 million times better than that available with light.
With quantum physics, we have a measurement capability that is 1 million times better than that available with light.
Such devices do exist. They are known, for example, as « inertial sensors using atomic interferometry ». The fields of application are very broad, ranging from geosciences (detection of oil slicks by measuring variations in the local gravity field) to life sciences (measurement of the electric or magnetic field emitted by a single cell) to inertial navigation (on Earth or in space).
In 2022, an article in Nature outlined the possibility of a gravity sensor of this type using the quantum behaviour of free-falling atoms to measure microscopic variations in the Earth’s gravity more accurately than ever before, in order to probe the structures beneath the Earth’s surface.
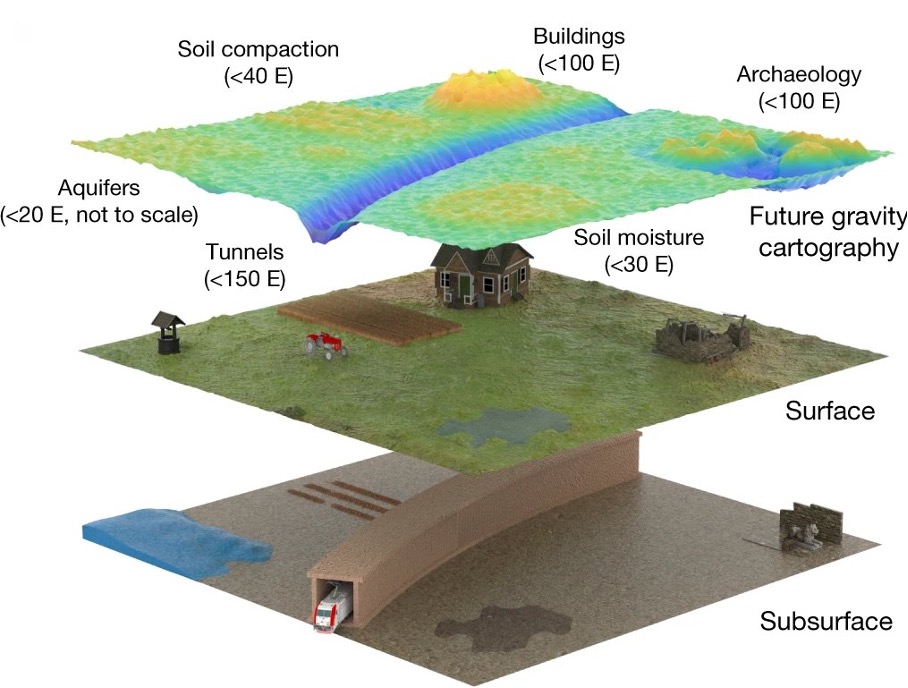
Quantum mechanics in the health sector
The pharmaceutical industry has also long since embraced quantum mechanics.
Medicines are molecules that bind to other living structures to provide health benefits for the patient. The way in which these molecules interact with each other is studied by a specific scientific field called “quantum chemistry”.
Before a therapeutic molecule is authorised for the market, it must undergo a series of tests and clinical trials that rarely take less than a decade. In order to target molecules of interest very quickly, there is a stage called « virtual high-throughput screening », where extremely complex algorithms test in parallel the ability of thousands of molecules to demonstrate the desired biochemical effect on the target.
This understanding of how an individual molecule chemically binds to other nanometric structures requires the development of digital simulation tools incorporating all the principles of quantum mechanics and chemistry, with the aim of delivering their results as quickly as possible, despite the colossal complexity of the calculations involved.
In France, a start-up called Qbit pharmaceuticals is developing new calculation methods combining neural networks, supercomputers and quantum computers to target the medicines of tomorrow ever more quickly and effectively4.