How synthetic biology could help degrade plastic waste
- 390 million tons of plastics were produced in 2021, the majority of which will probably be dumped back into nature.
- While plastics are a pollutant for most living things, some bacteria and fungi have acquired the ability to turn them into a source of energy.
- Synthetic biology is one of the tools that could be used to limit the pollution linked to the production and use of plastics.
- In particular, it proposes to apply engineering methods to molecular biology in order to “optimise living things”.
- Combining synthetic biology with these micro-organisms could be a solution for recycling plastics.
After a year of stagnation due to the pandemic in 2020, global plastics production has started to rise again: 390 million tonnes were produced in 2021, 90% of which was produced from fossil resources1. Once used, the majority is likely to end up as waste, adding to the 5 billion tonnes that had already accumulated in 2015, representing 79% of all plastic waste produced by humanity2. The environmental problems posed by these materials are numerous. Could micro-organisms help solve them?
Natural plastic eaters
Although plastics are pollutants for the vast majority of living beings, some bacteria and fungi have acquired the ability to turn them into an energy source. Found in the soil, the sea or the intestines of some animals, several species of micro-organisms can attack different types of plastics (PET, PP, PS, PE, PUR, PLA…) thanks to enzymes that alter these long hydrocarbon chains3. However, this does not mean that simply putting them in contact with the right plastic will make it disappear.
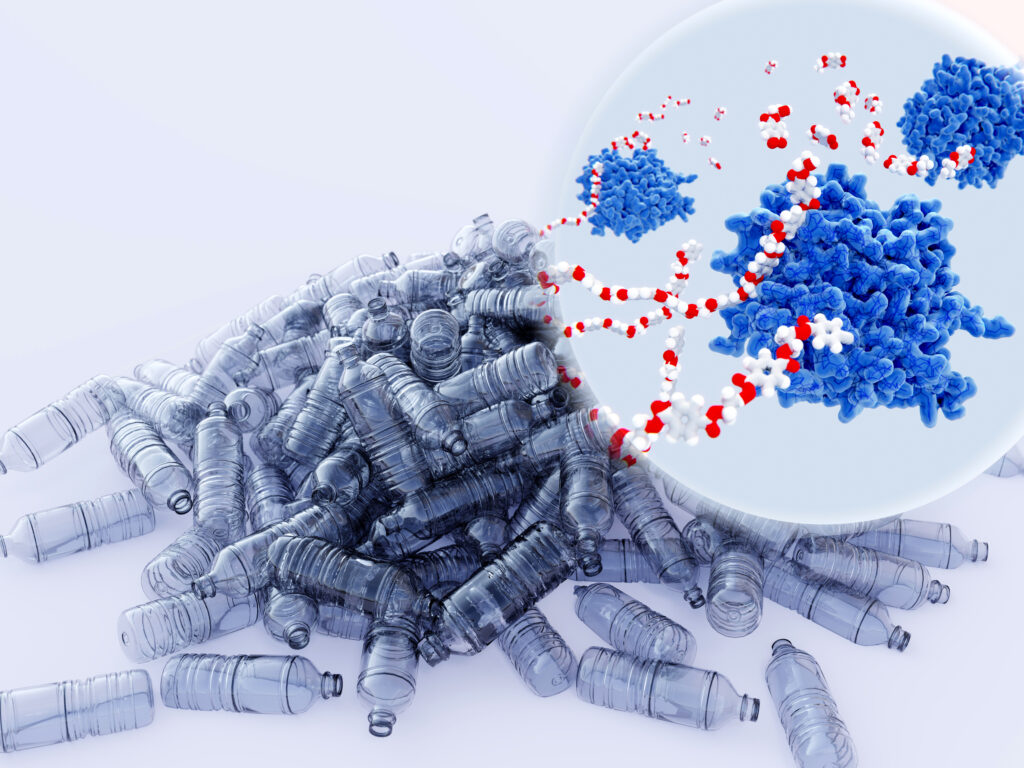
The process is most effective when the plastics have already been damaged, for example by UV light or chemical treatments, and when the micro-organisms are placed under optimal pH and temperature conditions4. However, each species is only active on certain plastics and being able to attack a material does not imply being able to degrade it completely. Even the strains that have attracted the most attention, such as Ideonella sakaiensis, a bacterium that breaks down PET into its constituent monomers5, have a major limitation: they need a few weeks or even months to degrade limited quantities of plastics. In other words, these plastic-eating micro-organisms are not an ideal solution for managing our pollution. But they could still be of great help to us!
Optimising what already exists
Progress in genetics, in terms of understanding the mechanisms involved, anticipating possible results, and developing molecular tools, now makes it possible to voluntarily modify genomes – in particular to make organisms synthesise certain types of protein. Many biological research projects now involve the production of cells or even organisms that over- or under-express certain genes. The genetic modifications that make it possible to obtain them are almost a craft, with each researcher making what they need. But this approach can be adapted to a completely different scale!
Since 2000, synthetic biology has proposed applying engineering methods to molecular biology. It considers genes and other DNA sequences (especially regulatory ones) as building blocks that can be optimised and combined in a metabolic engineering logic. The new biological synthesis pathways thus devised can then be implanted in cells, generally micro-organisms, which become small genetically modified production factories. This approach has its limitations, the main one being the chaotic complexity of living organisms. A synthetic pathway that seems optimal in theory does not always work in practice, once confronted with the reality of a cell’s environment. And the transition to a larger scale, which is essential for many applications, constitutes an additional level of difficulty: living systems often remain sensitive and unpredictable.
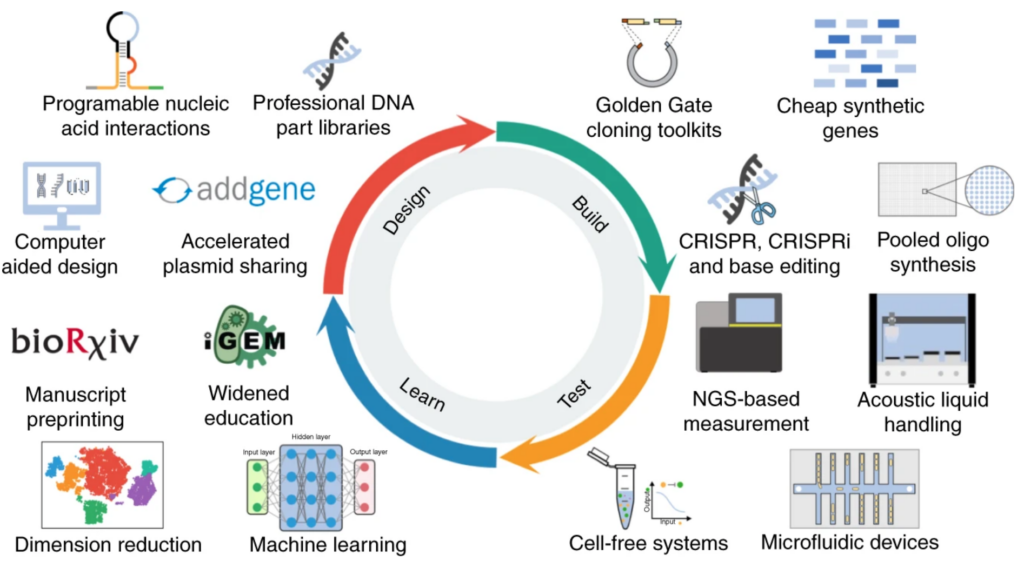
Nevertheless, in the last twenty years, synthetic biology has become much more than a theoretical vision. Many companies are investing in this approach, which has already led to the commercialisation of various products in fields as diverse as medicine, food, and materials7. Fuelled by biotechnological advances such as DNA synthesis, high-throughput sequencing, and new gene-editing techniques, supported by increasingly powerful computer tools and integrating new knowledge structured in increasingly rich databases, it seems reasonable to expect synthetic biology to produce breakthroughs8.
Attacking plastics
Microorganisms capable of degrading plastics may not be efficient enough to be useful on a large scale, but their study provides new ammunition for synthetic biology! Each enzyme discovered enriches the catalogue of tools available to design and optimise metabolic pathways. And the modification of these proteins after studying their structure sometimes makes it possible to obtain even more efficient versions of them, only a few years after their discovery910.
Unlike other recycling methods, which involve a loss of material quality, the biological degradation pathways allow the constituent monomers of the plastics to be returned. These can then be reassembled to produce a product equivalent to new, with no constraints on the colours or types of objects that can be manufactured. However, there are still problems to overcome. On the one hand, this deconstruction of plastics releases the additives added to these materials, which must be managed on their own. On the other hand, these approaches remain more expensive than production from fossil resources, and incentives will be needed to push manufacturers to implement them. Moreover, as yields are never perfect, the life cycle of plastics will not be infinite. Finally, even if they are no longer purely theoretical, these processes are still being developed!
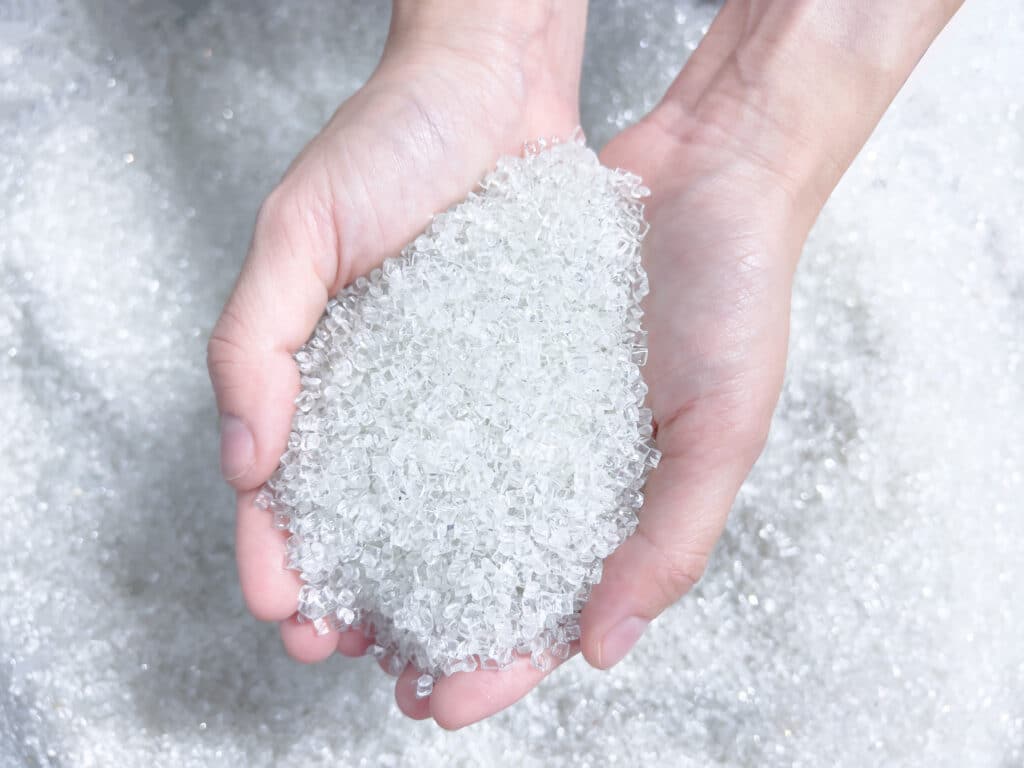
One of the pioneers of plastic degradation using synthetic biology is a French company: Carbios (https://www.carbios.com/fr/). It has developed a process based on an enzyme called LCC, identified in 2012 by Japanese researchers who carried out a metagenomic analysis (https://www.polytechnique-insights.com/tribunes/sante-et-biotech/la-metagenomique-comment-etudier-la-biodiversite-microscopique) of a compost11. Compared with other proteins capable of degrading PET, LCC was found to be particularly effective. Mutations were used to improve its activity and temperature resistance to produce monomers that effectively remanufactured PET to a quality comparable to new PET at a reasonable cost12. After setting up an industrial demonstrator13, the company continued its development with the construction of its first biorecycling site14.
Promises and limits
Beyond this example, the number of patents linked to the recycling of plastics and the development of alternative materials shows the dynamism of this sector15, which is of interest to both industry and fundamental research. Alone or in combination with other approaches16, synthetic biology is one of the tools that could make it possible to limit the pollution linked to the production and use of plastics.
However, this should not detract from the many issues raised by its use. Some are very clear, such as the management of additives, the improvement of yields, the optimisation of costs, the adaptation to different types of plastics and, generally, the difficulties of development and scaling up. Others touch on more delicate issues. Indeed, the micro-organisms produced by synthetic biology are genetically modified. This raises questions about the patentability of living organisms, but also about the risks of release into the natural environment. While the ability to degrade plastics is useful in a waste management context, it seems important to keep control of it.
In general, there is still a long way to go to achieve a reasonable use of plastics. Establishing a true circular economy is a challenge in itself17, reducing our dependence on these ubiquitous materials is another. While it is relevant to consider each tool to move in the right direction, no single tool will be sufficient to solve all the problems.