Artificial arteries to improve the study of disease
- The development of artificial arteries and vessels can help to better understand the fluid mechanics in arteries in order to better treat medical problems.
- This system is being deployed in collaboration with Lille University Hospital (CHU Lille) to measure the “Willebrand factor”, involved in thrombosis formation, which can result in blockage of blood vessels.
- Another artery model is used to represent microvascular diseases such as hypertension or Alzheimer's disease.
- These models make it possible to study this issue by representing a vessel in its complexity, with the different types of cells that make it up or that are associated with it in the brain.
Small winding paths or blood highways, vessels are essential elements of biology. Long considered as simple porous pipes by physiology, they turn out to be involved in complex physical and biochemical mechanisms that Abdul Barakat’s team is studying in the Hydrodynamics Laboratory (LadHyX).
We are developing artificial arteries and vessels to study the formation of biological phenomena, such as diseases, on different scales. The aim is to take into account a factor often neglected by modern biology: the fluid mechanics in arteries. We have thus devised two models, which can be adapted and applied to multiple medical questions.
From coronary artery disease…
The first is formed from a collagen hydrogel base forming a tube of 3 millimetres internal diameter and 4.5 millimetres external diameter. Smooth muscle cells and endothelial cells, which form natural arteries, are introduced into the tube. The result is an artificial vessel that closely resembles a coronary artery, which supplies the heart with oxygen. This model represents a very high-pressure flow regime with shear. It is intended to study coronary artery disease, also known as atherosclerosis.
In order to characterise the cellular response to the induced mechanical factors, it is possible to make use of high-resolution imaging systems, such as confocal fluorescence microscopy, as well as impedance sensors. By placing these sensors on the wall of our artificial arteries, we produce a true spatialisation that accounts for the cellular dynamics involved. This approach makes it possible to account for the dynamics of cellular responses or interactions between the different types of cells in the vascular wall.
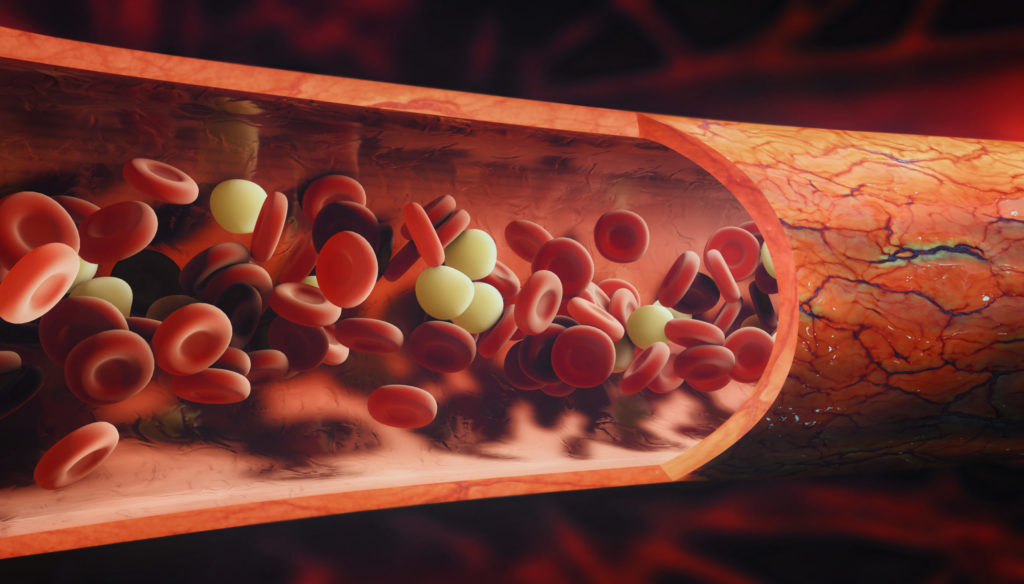
This system is, for example, deployed in collaboration with the University Hospital of Lille in order to measure the production and distribution of the Willebrand factor involved in the formation of thrombosis, which can clog blood vessels. It also enables the University of New South Wales in Sydney to study the effect of turbulence on arterial walls and the EFS in Strasbourg to study the formation of platelets in the blood.
At Ladhyx, this model is helping us to understand the healing of a vessel during the insertion of a stent, the small springs used to correct a vascular narrowing. The procedure can damage the endothelial cells that line the vessel lumen, creating a risk of “stenosis”, a collapse of the vascular walls. By anticipating the healing characteristics of each type of stent, we hope to avoid this phenomenon1.
… to microvascular disease
The second type of model, still based on collagen hydrogel, has diameters of 120 to 150 µm2 and allows the representation of microvascular diseases such as hypertension or Alzheimer’s disease. In the case of Alzheimer’s disease, this brain pathology is often considered as a disease caused by the abnormal behaviour of peptides (tau or β‑amyloid). However, this representation has not led to real clinical advances despite significant investment over the last few decades. Now, the idea is emerging that Alzheimer’s disease may result from a microvascular disorder. Laboratories have shown a correlation between this disease and cerebral hypoperfusion, i.e. abnormal blood flow in the brain.
In some animal models, it is possible to manipulate perfusion of the brain. In this way, it is possible to observe degeneration of neurons and an accumulation of the peptides associated with Alzheimer’s disease. We therefore suspect that an anomaly in mechanical factors participates in the development of the disease or may even initiate it. Our models make it possible to study this question by representing a vessel in all its complexity, with the different types of cells that make it up or are shared with it in the brain. We can even vary the cell composition to represent different biological situations.
In general, our models open up the study of the biological effects of mechanical forces. It is known that such factors can modify the expression of proteins. There is therefore a dimension of mechanical-biochemical interactions that we are seeking to explore.
From model to clinic
Advances in mathematical modelling, bioengineering and in silico medicine are leading to the development of increasingly efficient models of human pathologies. In an editorial for the latest Virtual Physiological Human conference 3, specialists showed that these latest generations of models have reached the clinic at several levels.
They can shed light on previously unknown aspects of physiopathology and thus open up new avenues of treatment. This is the case of the obstructive sleep apnoea model proposed by the team of Virginie Le Rolle and Alfredo Hernández at the University of Rennes, which analyses the coupling between respiration, metabolism and pulmonary mechanics.
Some of them allow individualised calculations according to a patient’s data and therefore improve the personalisation of management. The cardiac electrophysiology model combining MRI and ECG data developed under the direction of Gernot Plank, from the Medical University of Graz in Austria, is part of this approach.
Moreover, others have helped to anticipate and manage treatment regimes, such as the post-Caesarean section healing model proposed by Fernanda Fidalgo from the University of Porto. These are all examples how models and clinical practice converge.