Space exploration is becoming ever more ambitious with new missions to different planets in our solar system and beyond. My team at the LMD is contributing to this global effort by analysing the observations made by these missions and by developing global climate models to simulate the behaviour of extraterrestrial atmospheres using universal physics equations.
At the LMD most of my colleagues study Earth’s climate using satellite observations and numerical models that simulate its atmosphere. The aim is to model changes in climate and project what will happen in the future – say, 50 years from now. We have adapted our techniques and applied them to the atmospheres of the other planets in the solar system as well as to Titan (a moon of Saturn) and Triton (one of Neptune’s moons) and of course Pluto, formerly our ninth planet.
Numerical global climate models
Whether they are terrestrial or extraterrestrial, are a bit like a video game but they are based on physical equations that allow us to calculate all the phenomena around a planet – its clouds, winds, atmospheric circulation, dust storms, frost, snow. We then try to see if, simply on the basis of well-known theoretical equations, we can represent all these phenomena. This goal is very ambitious – sometimes we don’t succeed but, often, the models work astonishingly well. The results also allow us to better understand our planet’s atmosphere by reapplying lessons learned elsewhere. It is a bit like in medicine, in which animal models are used to better understand the human body.
We are currently dedicating much of our time to the planet Mars and are involved in a number of space missions including ESA’s Mars Climate Orbiter and Insight, an American mission, for which French colleagues provided the seismometer. This geophysical station is also a weather station. Again, we try to interpret the observations we make with our numerical models. We are also developing an ambitious EU-supported project called Mars Through Time, which involves using our extraterrestrial climate models to try and model the climate on Mars thousands, millions and even billions of years ago, when its orbit and axis of rotation were a little different and there were ice ages (recently) and even lakes and rivers (much longer ago) on its surface.
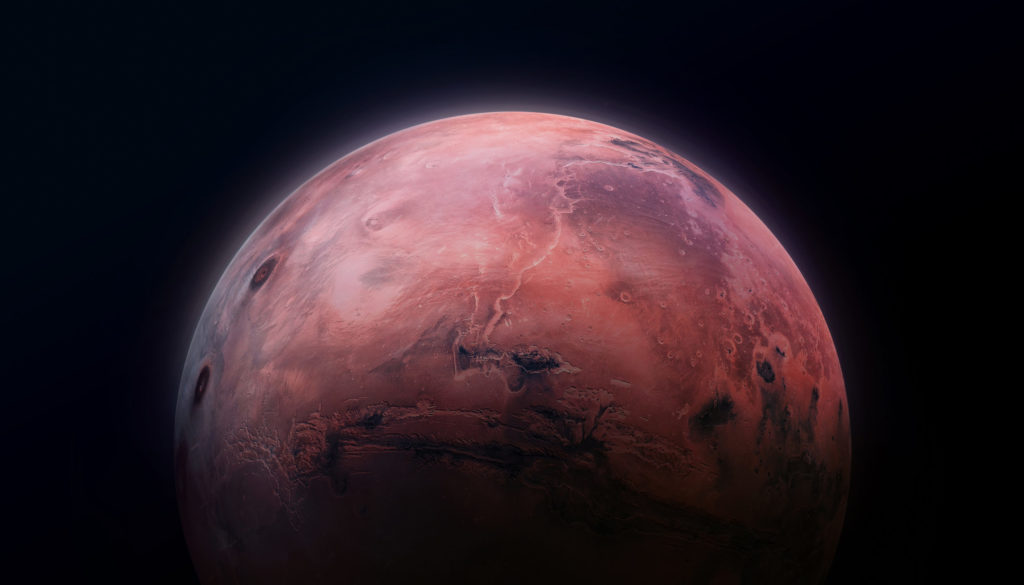
Surprises galore
For this and other projects, we recently applied a new computer programme called Dynamico, originally developed to study the Earth’s climate, to solve the equations of fluid mechanics to calculate atmospheric circulation – that is how depressions, anticyclones and winds evolve. When we applied the programme to Mars and Venus, we found that it did not simulate the situation on Venus very well. This is because subtle effects that the model neglects on Earth can be much stronger on other planets, which means that we sometimes have to improve the model by adding certain terms to the equations it contains. We encountered a similar situation a few years ago when applying our models to Mars. The extra term we added to our equations in this case not only helped us improve our model so that it better described the Martian atmosphere, it also helped us better simulate the monsoon in India when it was reapplied to Earth’s atmosphere. This may seem like a small detail, but it is an important result if we want to try and understand whether there will be a major drought or torrential rains in this area of the world in the future because of climate change. So, studying Mars’ climate has helped us to better understand Earth’s.
The results from these models, which are used by hundreds of research teams from around the world, are also crucial for preparing future space missions – especially those designed to land on the surface of a planet, or which will make use of the atmosphere to slow down their spacecraft. We are funded by space agencies and industry for these projects. The models can generally be trusted – which, incidentally, is very useful for convincing some climate sceptics. Indeed, it is amazing how well our models often represent what we observe. It is fascinating to see that a model will have predicted perfectly how, for example, the winds behave on a planet and that these predictions are confirmed by the data sent back from a real probe.
The situation is even more interesting when the model does not work. Sometimes this is because the situation is very complicated or “non-linear”, which means that the climate is extremely sensitive to such a such parameter. The model has to be finely tested and adjusted to account for this sensitivity. Most often, this implies that there is a physical phenomenon that we have not thought of and that is, in fact, present. This physical phenomenon may not be a process that acts directly on the environment by heating or cooling it, for example. It may be a feedback that drives the climate system into a certain operating regime, that is, a particular climate. We need to understand these physical systems, which have millions of degrees of freedom and combine many length and time scales, and appreciate what makes them enter certain states and function as they do. A real challenge and a beautiful 21st century physics problem for us to solve.
Références
- https://www.lmd.jussieu.fr/~forget/research.html
- https://www.sciencedirect.com/science/article/abs/pii/S0019103521004164
- https://www.sciencedirect.com/science/article/abs/pii/S0019103521003572
- https://www.sciencedirect.com/science/article/pii/S0012821X2100426X
- https://www.sciencedirect.com/science/article/pii/S0032063321001768
- https://agupubs.onlinelibrary.wiley.com/doi/10.1029/2021GL095453
- https://link.springer.com/article/10.1007%2Fs11214-021–00820‑z
- https://www.nature.com/articles/s41467-020–18845‑3
- https://link.springer.com/article/10.1007%2Fs11214-020–00716‑4
- https://gmd.copernicus.org/articles/13/707/2020/
- https://www.aanda.org/articles/aa/full_html/2020/02/aa37327-19/aa37327-19.html