The last few decades could be seen as a golden age for science. Indeed, it has the capacity to both describe and predict the behaviour of our world, allowing us to establish ways to apply that understanding to our lives: the genomic revolution, the quantum revolution, the reign of the Internet, and the triumph of relativity, to name a few. As science provides description and models, expectations grow for technology to provide the tools to tackle the big global challenges we are facing.
Although, recent past has also proven us to be in a time of post-truth, where ‘alternative facts’ or fake news run rampant and mistrust in science is rife. Often, the finger is pointed at the spread of social media as the culprit, but it must be said that it is not the only one.
Misinformation encourages a culture of suspicion with regards to scientific facts. However, simply pointing it out as such is not the remedy to reverse mistrust in science; confidence in science could be interpreted as a subjective ‘opinion’ and, as such, could drive the opposite effect further reinforcing doubt in a ‘denier’.
A scientific fact differs from opinion in that it must be described within a well-defined perimeter.
Therefore, this conundrum begs the question of what can be done. Are we able to re-establish trust in the scientific method and the pursuit of knowledge? Or are we doomed to fight against disbelief and unreasonable doubt that goes beyond the required level of critical thinking that is necessary for us to progress in our understanding of the universe?
Perhaps a good place to start is to consider the difference in normative status between ‘scientific fact’ and ‘opinion’. Apart from saying that science is what scientists are doing, which is part of its definition, scientific facts also have a well-defined perimeter of validity and are universal in this setup. This means they can be used to make predictions of which the confidence of such is based on replicable tests or experiments defined a priori and validated a posteriori. Last but not least, the hypothesis tested by experiments are objectively refutable – probably one of the main differences between ‘scientific fact’ with ‘opinion’.
Facts have well-defined boundaries
A scientific fact differs from opinion in that it must be described within a well-defined perimeter; one cannot talk about things “just like that” without a minimum of precision. For example, we can debate whether the sofa should go against the back wall or the left one. You may want it to go over there, because there is more natural light in that spot. Whereas I may want it to go over here where it is most practical to move around. Both are valid opinions, but neither are facts because we are both making our decisions based on our own (often, undefined) parameters. Moreover, the result wouldn’t be universal, meaning the results would depend on the owner of the sofa owner and his/her mood.
However, if we agreed that the sofa should be placed where there is most natural light – which we could define by prescribing an intensity distribution of light at specific wave lengths – then we have a defined, measurable parameter, which we can study in an objective manner. As such, using different methods we can research the precise location in the room where there is the most natural light based on empirical measures of UV rays, heat, time in the sun per 24 hours etc. Using those results we can define the perimeter of a scientific fact, mathematically model the precise, universal, sofa-owner-independent location in the room where the most natural light is found using the necessary analytical techniques.
Scientific facts can be used to make predictions
Once we have defined those parameters and the methods used to study them, the measures or experiments must be replicable. Replicability is defined as “obtaining consistent results across studies aimed at answering the same scientific question, each of which has obtained its own data1”. Hence, anybody should be able to reproduce the same results by applying the same protocol to a system. In the instance of the sofa, perhaps this would not be so difficult. When it comes to complex structures like living systems for instance, replicability is a huge challenge.
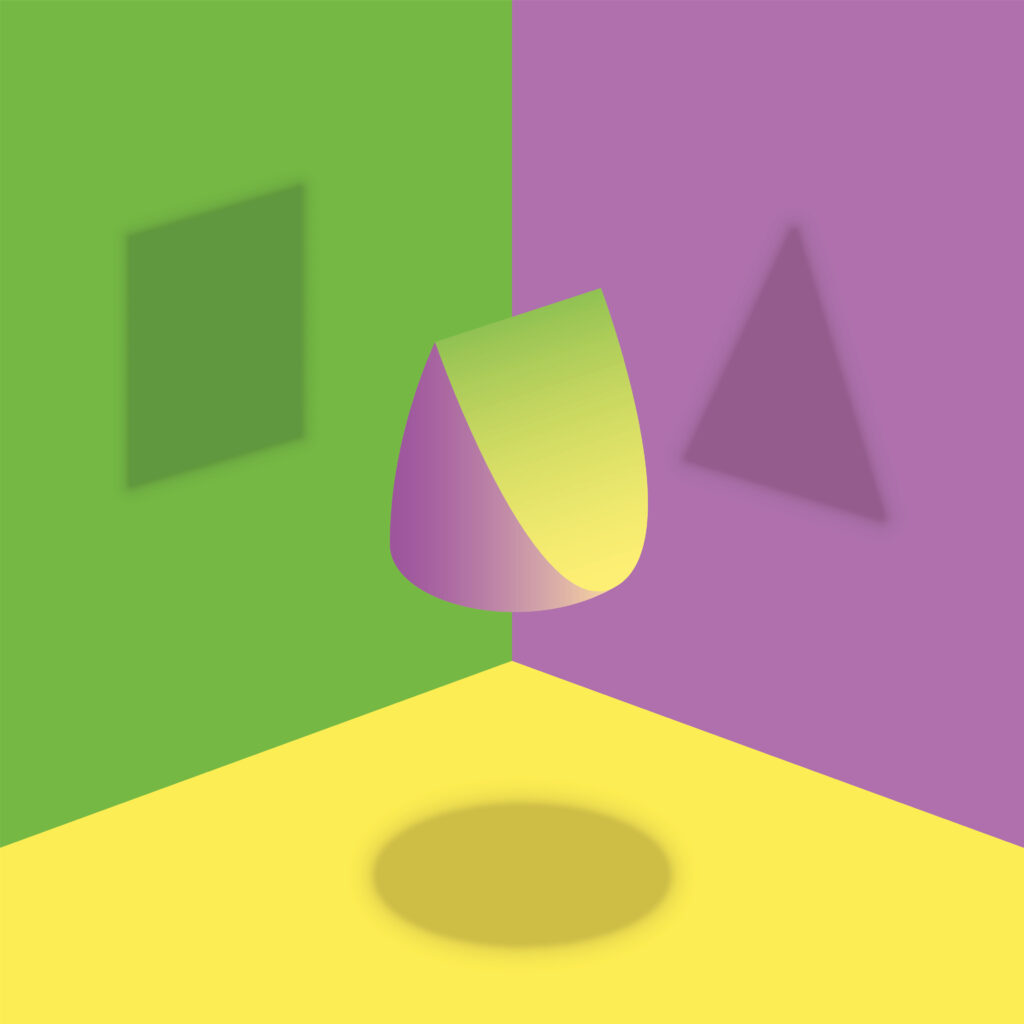
Moreover, in order to be ‘true’, the fact must be universal – the same laws of gravitation are in place whether you are in Paris, New York or at the North Pole. In fact, they are even the same laws if you are on Earth or Mars because, whilst you may not experience gravity in the same way yourself, Einstein’s theory of relativity still applies wherever in the universe you happen to be.
So, taking the first points into consideration, if scientific facts are the same everywhere and they are reproducible under the same conditions then they can be used to make predictions. If we know that every day the sun rises in the East and sets in the West, we can say with absolute certainty that it will do so tomorrow and every day that follows. And this will happen regardless of whether we believe it will: the sun does not, under any circumstances, care about our opinion of it.
Theories are more often refined than refuted
Once it is well-defined, has been tested reproducibly and can be used to predict outcomes, the only thing left is to test the limits of the scientific fact in question. In his motion theory, Isaac Newton posited that speed motion is always relative and time is absolute, whoever is computing it2. In one of his 19053 seminal papers, Einstein posited than the light speed c in a vacuum is absolute – implying in turn that time is relative. This non-intrinsic character of time is precisely one way of a possible ‘refutation’ of the theory, but, fortunately, has not yet been.
Even though Einstein’s relativity seems to bury Newtonian physics, he didn’t actually refute his predecessors’ theories. Rather, he refined them. Both Newton and Einstein were essentially right: Newton’s physics is right for ‘slow’ speeds (bearing in mind that even a hypersonic rocket has a slow speed in this context!) but not for speeds close to c (the speed of light). For slow speeds, both Einstein’s and Newton’s theories coincide. Einstein simply offered a more thorough explanation of the universe.
Another example would be genetics. When Mendel was studying heredity in pea plants, he knew that characteristics could be passed down through the generations in a species. We then learnt of the existence of DNA and that heredity is contained within the genes that parents transfer to their offspring. So, for a while, scientific fact had been that our genetic inheritance was defined solely by our DNA that was wired at birth.
Even though Einstein’s relativity seems to bury Newtonian physics, he didn’t actually refute his predecessors’ theories. Rather, he refined them.
More recently, we discovered epigenetics: the existence of molecular switches capable of turning genes on or off in a process that can happen at any point over the lifespan of an organism. Thus meaning that experiences can influence gene functions by making small adjustments to our DNA and, on top of that, these ‘acquired’ modifications can be transferred to our offspring through the generations. Again, the role of DNA in heredity was not refuted; instead, it was our understanding of the bigger picture that matured.
These examples show the fundamental importance of the scientific questioning driven by the fruitful ‘collective doubt’, opposite to peremptory assertions often surrounding opinions or worse ‘alternative facts’.
Doubt is healthy, mistrust is not
It is this ‘collective doubt’ that scientists share with one another, which allows that refining process to happen. Questioning one another, challenging the methods used and adding new information from other sources helps scientific facts flourish in a way that becomes extremely difficult to refute. Hence, rather than weaken scientific fact, scientific doubt actually serves to strengthen it. Therefore, in the end, it is actually very rare that accepted scientific fact is entirely thrown out to the trash when faced with new findings. Rather, scientific facts tend to be refined. We redefine the outlines and learn more about the parameters or methods used, allowing us to chisel a clearer image of the truth like that of a pixelated computer screen, which becomes sharper as we add pixels. After all, the goal of science is to persistently improve the definition of the image we have of the universe.