Laser-generated plasmas can be used to accelerate particles that can then be used to create short, bright pulses of X‑rays and gamma rays. The Laboratoire d’Optique Appliqué (LOA) and its spin-off company SourceLab are developing a device to use these sources for the non-destructive testing of materials using X‑rays. This cutting-edge technological innovation could enable researchers to detect and size defects in objects with a resolution of a few tens of microns, something that is still inaccessible with conventional systems.
An increasingly accessible technique
Particle accelerators have enabled us to make the most important discoveries in physics, but they collide particles at ever higher energies, pushing existing technologies to their limits. Indeed, acceleration facilities, such as the Large Hadron Collider at CERN, are getting larger and larger and are becoming inaccessible to many researchers.
In recent years, “plasma wakefield accelerators” have emerged as a promising alternative. These devices use a pulse of energy to create an electric field wave in a plasma (a gas transformed into a cloud of electrons and ions), rather like the wake in water created by a boat. If a group of particles is properly synchronised, it can “surf” on this wave and be accelerated much faster than in a conventional accelerator. However, it is not easy to create this energy pulse.
One way to do this is to send extremely short and intense laser pulses into a gas. The front of the pulse, which lasts only a few femtoseconds (10-15 s), immediately ionises the atoms in the gas and is so intense that it pushes the electrons out of its path, forming an empty cavity of electrons in its wake. Some electrons in the wake of the pulse are accelerated by the wave of positively charged plasma ahead of them, just like a surfer on the wave behind the stern of a boat. The electrons “surfing” on this wake can be accelerated to speeds close to the speed of light.
What are these applications?
We study this phenomenon at LOA. It allows laser-plasma accelerators to achieve acceleration forces up to a thousand times greater than those achieved by the most powerful machines available today. Our laboratory is a pioneer in this field, and we have been working on this subject since the mid-2000s. Together with other teams around the world, we have developed the technology to the point where we created the Laplace Laser Plasma Acceleration Centre in 2022 to better understand the mechanisms involved and develop applications.
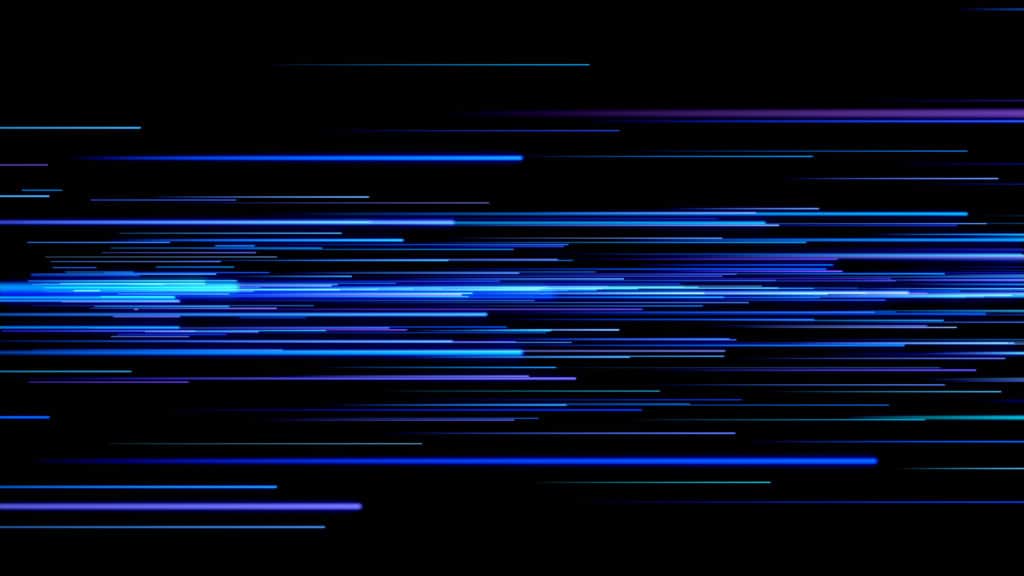
The first is radiography, particularly in the field of non-destructive testing, a set of techniques that are used to check components and identify defects in a material without destroying it. The size of the particle packets supplied by laser-plasma sources is on the order of ten microns, making it possible to probe components at high resolution, for example those important to the nuclear and aeronautical industries. Initial laboratory results indicate that it would be possible to monitor the appearance of cracks as small as 100 microns in parts such as landing gear. This is ten times smaller than the detection limits of current equipment.
So, it is a simple way to see if there’s a crack in a piece of steel, for example, without having to slice it, which would mean replacing a potentially very expensive part.
What we are interested in here is the conversion of electron beams into a highly energetic X‑ray beam – using the technique known as braking radiation. In practice, we send the electron beam into a millimetre-thick sheet of a fairly dense material, such as titanium. The electrons are slowed down, or braked, and the energy lost by their slowing down is converted into X‑rays whose maximum energy corresponds to the energy of the initial electrons with a very broad spectrum. This is a fairly simple and relatively efficient method of producing X‑rays from an electron beam.
Materials radiology
The size of the defects we can detect and monitor is largely determined by the size of our accelerator source. Laser-plasma acceleration is a good solution in this respect since we start with a very small source size. This means that the size of the X‑ray beam will also be small. In fact, it is a bit larger than the electron beam, because the electron beam has a large spatial divergence. As the beam diverges, the diameter of the electron beam on the converter is larger than its size at the plasma exit. Typically, if you have a micrometric source size, you end up with a beam size of the order of tens of micrometres, typically 30 to 100 microns, which is still a good order of magnitude.
We are a bit like medical radiologists, but our “patients” are materials. When we pass X‑rays through a material, they will be less absorbed in the region of a defect. We can image that defect in exactly the same way as a radiologist does for bone fractures. Since our X‑rays do not pass through the human body, but through pieces of concrete or steel, this means that we need much higher energy rays than those used by radiologists.
Références :
- V. Malka, C. Thaury, S. Corde, K. Ta Phuoc and A. Rousse ; Accélérateurs à plasma laser : principes et applications Reflets de la physique 33, 23–26 (2013)
- A. Ben-Ismaïl, O. Lundh, C. Rechatin, J. K. Lim, J. Faure, S. Corde, and V. Malka Compact and high-quality gamma-ray source applied to 10 μm-range resolution radiography
- Applied Physics Letters 98, 264101 (2011); https://doi.org/10.1063/1.3604013