It is a problem that has plagued biologists for over fifty years: how is the helix structure of proteins and DNA formed? Whilst various experimental techniques do exist to determine the precise three-dimensional structure of biomolecules, measuring their dynamics, which happen in the range of several femtoseconds (10 x 10–15 seconds) to seconds, is still an experimental challenge. This area of research is being explored by a team of French researchers, led by Pascale Changenet and François Hache at the Optics and Biosciences Laboratory (LOB) at École Polytechnique, using time-resolved circular dichroism.
What is circular dichroism?
Pascale Changenet. It is a technique based on chiral compounds, which absorb polarised light differently depending on whether it is right or left-handed circular. A compound is chiral when it is not superimposable on its image in a mirror. Such objects can therefore exist in two forms, called enantiomers in the case of molecules. “Chirality” is an important component of living organisms that can be found everywhere at different scales. Our hands are examples of chiral objects. Proteins and DNA are chiral molecular assemblies made up of elementary building blocks (amino acids and nucleotides) which are themselves mostly chiral. Using circular dichroism, it is possible to characterise their spatial helical arrangement, especially when they are in equilibrium, in solution.
However, circular dichroism is still underdeveloped for dynamic (or non-equilibrium) measurements, particularly at ultra-short times. This requires the use of lasers delivering femtosecond pulses and the so-called “pump-probe” technique. A first intense laser pulse, the pump, is used to ‘perturb’ the biomolecules, while a second, less intense pulse, the probe, is used to observe the response of the system to the light perturbation. It is a bit like the flash that precedes the taking of photographs a few moments later. By controlling the delay between the arrival of the pump and probe pulses in the samples, it is possible to measure the sequence of events triggered by the pump with a temporal resolution limited by the duration of the pump and probe pulses. In addition, by precisely controlling the circular polarisation of the probe at each pump-probe delay, we can trace the film of the conformational change of biomolecules over time.
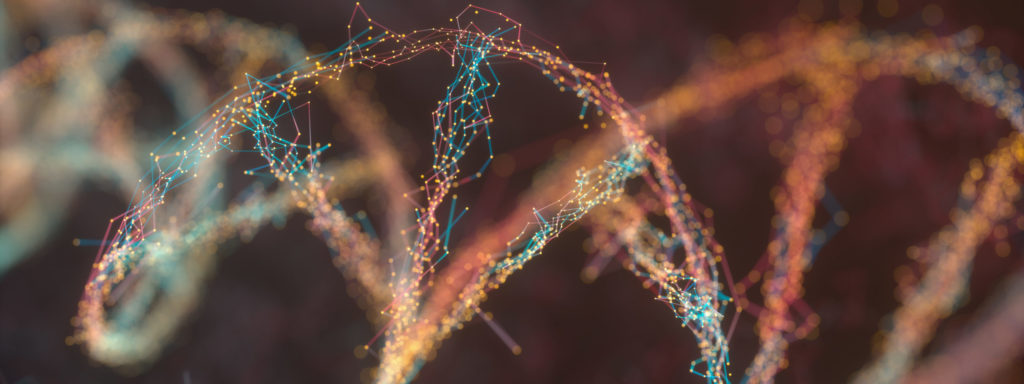
What are the advantages and disadvantages of time-resolved circular dichroism compared to other techniques?
The main advantage of circular dichroism is that it can access the conformational dynamics of biomolecules down to extremely short time scales, which is, for example, not possible with other comparable techniques such as nuclear magnetic resonance (NMR). Although it is possible today to make time-resolved X‑ray diffraction measurements with very short time resolution, this requires the use of large instruments such as synchrotrons or free electron lasers (XFEL) which are very expensive and have limited access.
While circular dichroism gives less precise information on the structure of biomolecules than X‑ray diffraction, our experiments use commercial laser sources and can be performed directly in our laboratory. The principle of these measurements is extremely simple. However, they require the ability to detect extremely small variations in light signals of the order of 1/10,000, which is probably why very few people use it at the moment. With constant progress in the development of laser sources and optics, these experiments are set to become increasingly accessible.
François Hache. Our team is a pioneer in the development of circular dichroism measurements at very short times. The advantage of these measurements is that they do not require specific modifications of the samples studied and require very small quantities of proteins or DNA, compared to X‑ray diffraction measurements which require the crystallisation of samples and are destructive.
How have you responded to the rapid advances in artificial intelligence for predicting the conformation of biomolecules?
PC. It is becoming possible to reliably predict the three-dimensional structure of proteins from their sequences, especially with the recent development of the Alpha Fold 2 algorithm. However, the function of biomolecules is not only linked to their three-dimensional structure, but also to their dynamic changes. Measuring and modelling these dynamics – spanning time scales of several orders of magnitude – is still a major challenge in modern biophysics.
What are the potential clinical applications that could result from your research?
FH. Our studies focus on biomolecules in solution in vitro. It is difficult to envisage studies of this type in cells, tissues or even in vivo. Our work is primarily aimed at understanding how helices are formed in proteins or DNA and identifying the determining environmental factors, such as temperature, pH or the nature of the ions. Understanding the effects of the interaction of certain molecules specifically targeting DNA on its structure is an avenue that we are also exploring to provide input for new research avenues in pharmacology or medicine.