The idea of using thorium as a nuclear fuel was largely abandoned in the past because, traditionally, nuclear power was linked to military nuclear research and development – and both uranium and plutonium were used to make atomic bombs. For power generation, however, thorium could have real advantages and several countries are investing in this chemical element. The metal could be used in molten salt reactors, one of the new generation designs in which the reactor coolant and the fuel itself are a mixture of hot molten salts. These types of reactors can reach very high temperatures, which greatly increases the efficiency of electricity production.
The problem, however, is that more than 400 nuclear power plants in operation around the world use mainly uranium (U) as fuel. Although this element is abundant, less than 1% of the uranium on Earth is U‑235, the isotope of uranium that is fissile. The rest is U‑238. The U‑235 contained in uranium must therefore be concentrated and then enriched in complex and expensive processes.
And that is not all: the fission of U‑235 produces highly radioactive waste that must be handled carefully and then stored in a safe place for extremely long periods of time. This waste also contains a type of plutonium that can be used to make nuclear weapons.
Thorium reactors around the world
China has constructed an experimental thorium reactor at Wuwei, on the outskirts of the Gobi Desert1. Thorium has been tested as a fuel in other types of nuclear reactors in countries including the US, Germany, the Netherlands and the UK. It is also part of a nuclear programme in India because of the natural abundance of the element in that country. In France, studies are being carried out by the CNRS, which is developing a project called MSFR (for Molten Salt Fast Reactor), using thorium 2.
Four times more abundant than uranium
Thorium (Th) was discovered in 1828 by the Swedish chemist Jons Jakob Berzelius, who named it after Thor, the Norse god of thunder. It is a slightly radioactive metal found in rocks and soils and is quite abundant in the Earth’s crust. Indeed, its main isotope, Th-232, is about four times more abundant than U‑2383 and as abundant as lead. The amount found in the United States, for example, could meet that country’s energy needs for a thousand years without the need for the enrichment required for uranium-based fuels.
The rare earth phosphate mineral, monazite, contains the most thorium – up to about 12% thorium phosphate 4 Monazite is found in igneous and other rocks and the world’s monazite resources are estimated at about 16 million tonnes, of which 12 Mt are found in heavy mineral sand deposits on the south and east coasts of India.
Th-232 is of interest for nuclear power generation because it can easily absorb neutrons and transforms into Th-233. This new isotope emits an electron and an antineutrino within minutes to become protactinium-233 (Pa-233). This isotope, in turn, transforms into U‑233, which is an excellent fissile material. Indeed, the fission of a U‑233 nucleus releases about the same amount of energy (200 MeV) as that of U‑235.
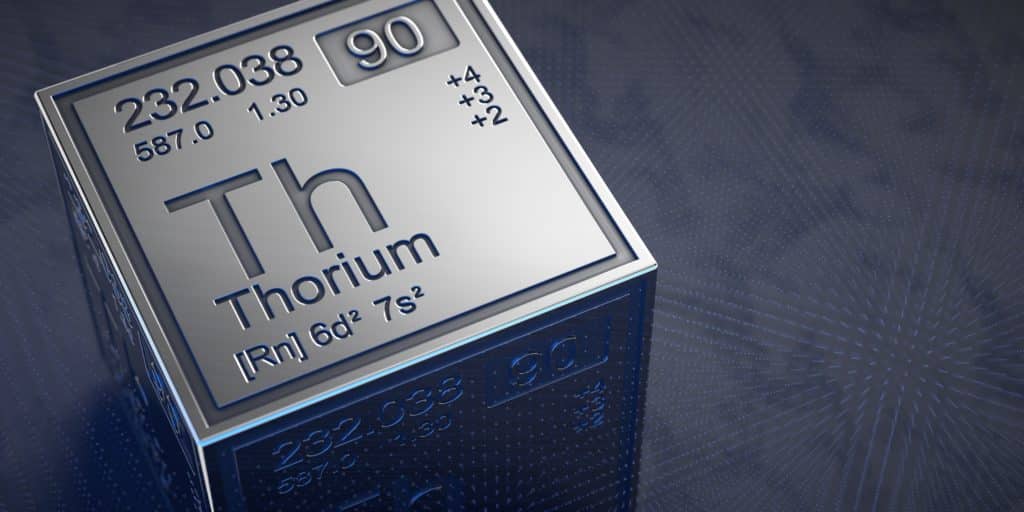
The problem of cooling
In conventional reactors, uranium is stored in solid fuel rods, which are cooled by huge amounts of water. Without this cooling, the rods would melt, releasing dangerous radiation. The thorium would undergo its reactions in an entirely different type of reactor, called a molten salt reactor (MSR), containing a mix of fluoride salts in which the nuclear fuel is melted. This type of reactor does not need to be built near watercourses, as the molten salts themselves serve as a coolant.
This means that the reactors can be installed far from coastlines, in remote and even arid regions. These reactors cannot ‘meltdown’ in the conventional sense either and, in an emergency, the fuel can be quickly drained from the reactor. MSRs deploying thorium are also safer because they operate at pressures close to atmospheric pressure.
Like uranium, thorium absorbs neutrons, as mentioned, but unlike uranium, it does not release more neutrons to perpetuate the nuclear chain reaction. This reaction starts when a uranium atom is hit by a neutron, releasing energy that causes more neutrons to be ejected from the uranium atoms, starting the cycle again. By reducing the number of neutrons injected into the fuel, it is the thorium itself that limits the rate of the nuclear reaction.
R&D investments needed
The use of thorium as a new primary energy source has been an attractive prospect for many years, but extracting its latent energy value in a cost-effective way is a challenge. The development of new thorium-fuelled nuclear power plants will therefore require significant research and development and testing – something that may be difficult to justify given that uranium is relatively cheap and abundant.
Another disadvantage is that thorium is ‘fertile’ and non-fissile, so it can only be used as a fuel in combination with a fissile material, such as recycled plutonium, as a conductor to maintain a chain reaction (and thus a supply of excess neutrons).
The U‑233 produced at the end of the cycle is also difficult to handle, as it contains traces of U‑232, which actively emits gamma radiation. While some researchers support the use of thorium as a fuel because its waste is more difficult to turn into atomic weapons than uranium, others argue that risks remain5.
On the bright side, there is less plutonium produced overall during reactor operation. Some scientists say that thorium reactors could even help deplete the tons of plutonium we have created and stored since the 1950s.