The EU has set ambitious Greenhouse Gas (GHG) emission reduction targets, aiming at carbon neutrality by 2050, with a milestone of ‑40% emissions by 2030. Hydrogen is a major pillar of this strategy, and its share in the European energy mix is expected to grow from less than 2% (including use as a feed stock) in 2018 to ~14% in 2050 1. Therefore, as of 2030, European hydrogen demand is expected to increase significantly (+340 TWh between 2015 and 2030), with industry (+ 164 TWh) and mobility (+70 TWh) being the main growth contributors. Economic competitiveness and carbon footprint of the hydrogen supply is crucial to ensure its suitability for these key applications.
The hydrogen economy
Even though it is currently more expensive, there is economic room for hydrogen electrolysis; the only credible way to produce sustainable and carbon-free hydrogen. For that, the market will need to find areas of cost competitiveness in the form of “semi-centralised” models as mid-to-large scale mobility hubs capable of producing 10–50MW. Attractive green hydrogen costs are expected to be achieved by 2030, in the 2–3$/kg range, if coupled with best cost and capacity factor renewables, such as Solar PV around the Mediterranean Basin or Off-Shore Wind near to North Sea Coasts.
For industrial applications, costs of “blue” hydrogen are expected to match with current “grey” processes, which are currently the lowest 2. As these production methods still produce CO2, they must be combined with high CO2 capture, expected to reach up to 90% as carbon capture and storage reaches technical maturity. Wherever its implementation is feasible such as the North Sea (where TotalEnergies, Shell and Equinor are deploying major investment) “blue” hydrogen it will be able challenge “green” (or CO2-free) hydrogen costs and emissions. In other areas, large green hydrogen projects like Masshylia (50MW) or Port-Jérôme (200MW) appear to be the best options.
Distributed hydrogen production, technically consistent for light mobility applications, is nonetheless structurally hampered by limited scale and high grid power supply costs and requires consequently public subsidies.
Hydrogen vehicles
The EU has laid out a Hydrogen Roadmap plan that includes the deployment of 45,000 fuel cell trucks and buses (FCEB) on European roads by 2030, positioning hydrogen as a linchpin in the decarbonisation of public transportation. Indeed, heavy-duty road transportation (either long-haul trucks or urban buses) appears to be one of the most promising candidates to leverage hydrogen mobility hubs in the mid-term.
From a technical standpoint, fuel cell (FC) heavy-duty vehicles are a technically attractive solution as they use a set of mature technologies (fuel cells, storage tanks, etc.). As such, they have been extensively validated in testing programs such as Jive 1 / Jive 2 for buses in Europe, while most alternatives to ICE (internal combustion engines) like batteries are still facing technical challenges including limited autonomy, long charging times and payload limited by weight, for example.
Economically, purchasing costs of FCEV are also expected to decrease over the next decade reaching 120k€ for a FC truck and 325k€ for a FC bus by 2030 – compared to 300k€ and 650k€ today, respectively. Coupled with competitive hydrogen price, these cost improvements will put FCEV TCO at par with traditional vehicles.
Even with “grey” or “blue” hydrogen, which still emit CO2, FCEV vehicles do bring overall improvements in CO2 emissions compared to current models, about 10–15% if powered with “grey” hydrogen, then up to 80% less with “blue” hydrogen. Also, they do so whilst staying on the same level as the best EURO 6 trucks in terms of NOx emissions.
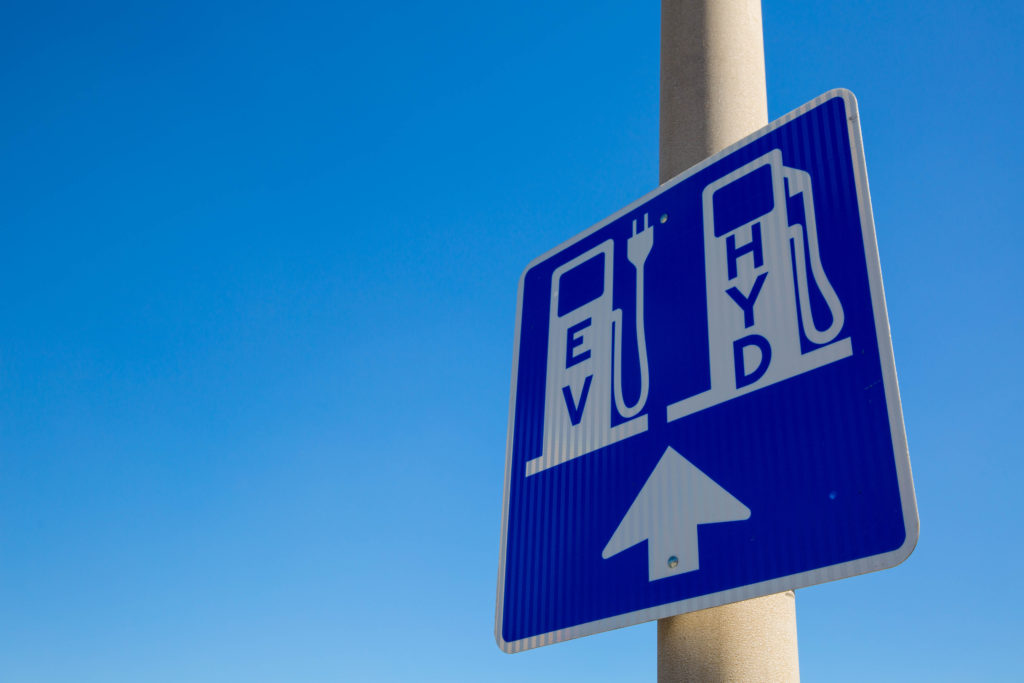
However, there is no such thing as a free lunch. In addition to the development of electrolysers (or CCS systems if relevant), the deployment of heavy hydrogen mobility requires rapid – albeit, realistic – end-to-end value chain activation. This includes standardisation of distribution infrastructure – today a major part of hydrogen delivered costs – which is a key challenge to rapidly gain scale and widely deploy refuelling stations at strategic locations, such as logistic hubs along Pan-European freight key routes (“TEN‑T”).
Deployment costs are expected to be sizeable, too. For instance, switching 10% of long-haul freight transport in 6 Key EU countries (France, Germany, Italy, Benelux) – equivalent to ~20,000 trucks – would require €2bn to €3bn CAPEX for refuelling infrastructure. The funds would create 600 refuelling stations, and as much for hydrogen liquefaction and transportation equipment in the case of remote hydrogen production. However, such investments are of reasonable magnitude when compared to governmental announcement for hydrogen, and bold moves are also expected from private players (industrial gas producers, highway operators, truck manufacturers, logistics, O&G players, power & gas utilities).
Hydrogen in space
Terrestrial mobility will however not be the only hydrogen application to witness major transformations in the next decade. Indeed, Lunar and Mars exploration are experiencing a resurgence of interest globally, as NASA awarded SpaceX a $2.9bn contract to build a Moon lander by 2024 and Elon Musk recently reiterated his ambition to land manned spacecraft on Mars before 2030.
The huge difference in the energy required to launch from Earth and from the Moon is pushing industrial players to consider refuelling points (e.g. EML‑1, NRHO) in cis-lunar space with an energy source that will be mined and processed on the Moon. Being able to produce economically space propellants (LH2, LOX) on the moon could foster deep-space exploration programs.
Therefore, new frontiers are on the rise for hydrogen electrolysis. To produce space propellants on the Moon, the whole value-chain must be replicated in situ: regolith mining, water separation and processing, logistics and transportation, robotic operations, communication systems and power plants. Necessary technologies having been developed or are currently in development. Several players are currently addressing this nascent value chain in a rather scattered way, an integrated approach being however fundamental as isolated initiatives are unlikely to deliver tangible results.
As of 2030, if the current ambition is achieved, a ~240-tonne-per-year propellant market could emerge at the future Gateway station (NHRO), with a foreseen economic viability if space launch prices from Earth do not plummet significantly and if currently contemplated CAPEX levels are kept under control. Scale will play a major role, to absorb major one-shot R&D costs, through the development of complementary uses such as rovers and human life support.